Galaxy Clusters and the Euclid mission
Gianluca Castignani1,2, Giulia Despali1,2, Carlo Giocoli2,3, and Lauro Moscardini1,2,3 on behalf of the SWG Galaxy Clusters of the Euclid Consortium
1Dipartimento di Fisica e Astronomia “Augusto Righi”, Alma Mater Studiorum – Università di Bologna; 2INAF – Osservatorio di Astrofisica e Scienza dello Spazio di Bologna;
3INFN – Sezione di Bologna
Contribution presented by Bruno Marano
Abstract
The Euclid mission is one of the major ongoing space missions undertaken by the European Space Agency, recently launched on July 1st, 2023. Euclid will map over 40% of the sky enabling the observations of 1.5 billion galaxies. These will give the possibility to reconstruct the 3-dimensional distribution of galaxies in our Universe up to the earliest epoch, and thus shed light on the elusive dark components, which contain the vast majority, but still unknown, of the mass-energy budget. In parallel, the Euclid data-set will allow the detection of thousands of galaxy clusters, the most massive gravitationally bound structures, which originate at early epochs from primordial perturbations and grow in mass through the entire cosmic history of the Universe. By modeling their distribution in time and space, galaxy clusters will be used to measure key cosmological parameters, such as dark matter and dark energy density parameters, as well as to study galaxy evolution in dense environments, which are very rich of astrophysical phenomena.
1. The Euclid mission
Euclid is one of the major forthcoming collaborative space missions undertaken by the European Space Agency (ESA, [12, 17]). The Euclid spacecraft was launched on July 1st, 2023 from the Cape Canaveral Space Force Station in Florida, with the Falcon 9 reusable two-stage rocket designed and manufactured by SpaceX1, see Fig. 12. The satellite will orbit at the second Lagrange point L2 in the frame of the Earth-Sun rotating system [23]. The L2 point allows Euclid to minimize the impact of thermal noise, which is key for infrared observations such as those envisaged by such an unprecedented space mission. The spacecraft indeed carries two main instruments: the visible and near-infrared imaging instrument (VIS, [7]) and the Near Infrared Spectrometer and Photometer (NISP, [19]). These two instruments are complementary and are designed to provide photometric and spectroscopic data over a wide field-of-view with unprecedented high accuracy and precision.
Euclid will address fundamental questions in modern cosmology, such as the nature and properties of dark matter and dark energy, the geometry of the space-time and the validity of General Relativity on cosmic distances, the formation and evolution of large-scale structures, and the formation and evolution of galaxies over cosmic time.
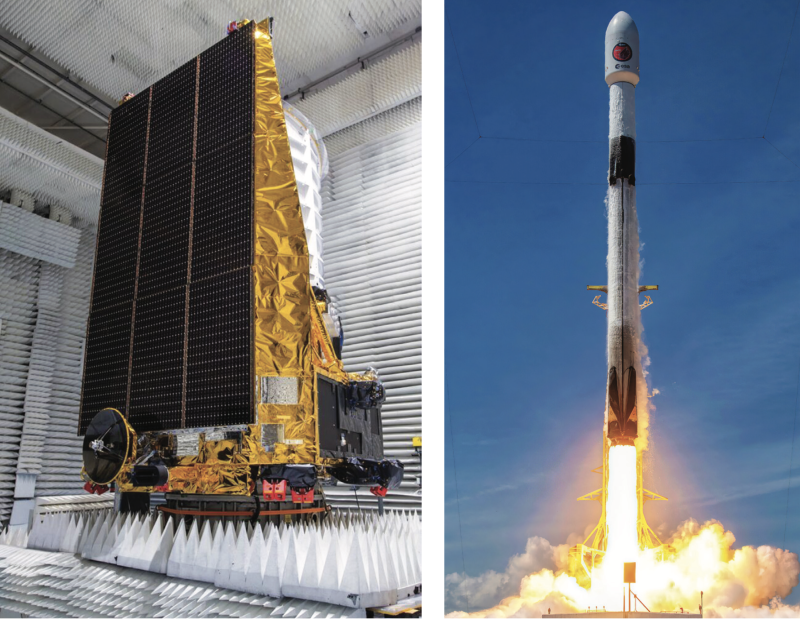
Fig. 1. Left. Euclid satellite at Thales Alenia Space in Cannes, France, on February 8th, 2023. Credits: ESA – M. Pédoussaut Right. Launch of Euclid from Cape Canaveral on July 1st, 2023. Credits: SpaceX.
The major goal of the mission is to unveil the mysteries underlying the dark Universe. Euclid will achieve this ambitious goal by observing during its ∼ 7 years mission about 1.5 billion galaxies over about 40% of the sky, up to the primordial Universe. By analyzing the distribution in shape and space of these galaxies Euclid will shed light on the long-standing debated nature of dark matter and dark energy.
Dark matter in particular makes up about 27% of the total mass-energy content of today’s Universe. This fraction largely surpasses that of the ordinary, baryonic, matter, which indeed comprises only 4-5% of the total mass-energy budget of the present-day Universe. Nonetheless, dark matter does not interact via strong or electromagnetic forces, which makes it very elusive and difficult to detect with current observatories and laboratories. However, dark matter manifests itself via gravitational effects on visible matter, such as in the case of flat rotation curves of disk galaxies, X-ray halos due to the emission of hot gas in elliptical galaxies, and in the case of clusters of galaxies, which show spectacular weak and strong lensing features. The nature of the dark energy is even more unclear. Dark energy accounts for about 68% of the total mass-energy content of the Universe, and it is believed to be responsible for the accelerated expansion rate of the Universe.
The path of the light originating from extra-galactic sources at cosmological distance is ultimately impacted by the intervening matter, and in particular by the dark matter and dark energy components. Euclid aims to study this effect to ultimately understand how the geometry of the Universe depends on cosmic time. To this aim, the Euclid mission will use a variety of complementary techniques. One of these primary cosmological probes is weak gravitational lensing, which aims at measuring small distortions of the shapes of distant galaxies due to the gravitational bending of light by intervening matter [10]. The weak gravitational lensing is therefore an effective tool to weight the dark matter contribution. The other primary probe is represented by galaxy clustering, which is a technique that measures the three-dimensional spatial distribution of galaxies. This measure, as a function of cosmic time, is then related to the total matter distribution and to the dark energy density [8, 27].
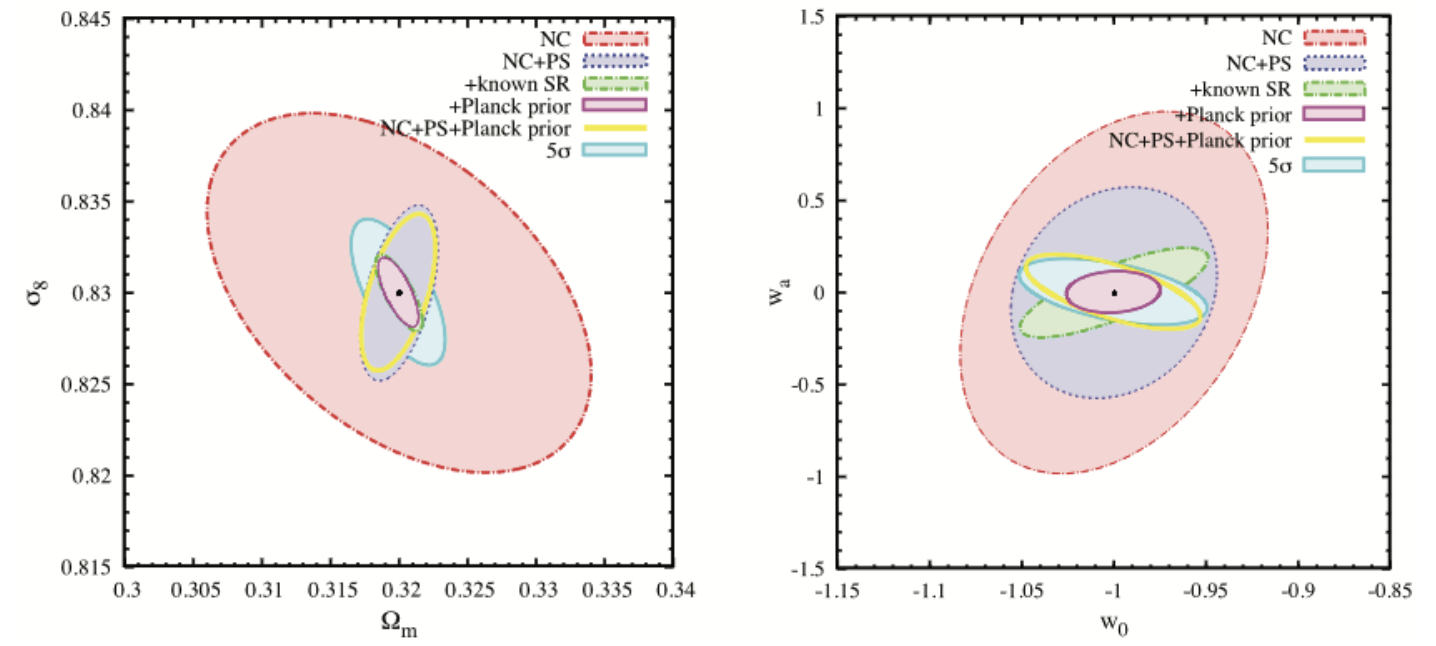
Fig. 2. Forecasts of key cosmological parameters that will be constrained using Euclid clusters. Credits: [25].
2. Galaxy clusters as cosmological probes
The combination of the information obtained with the primary cosmological probes outlined above and the complementary ones will ultimately enable to measure key cosmological parameters at percents accuracy (Fig. 2, [25]). The exploitation of Euclid data will thus allow us to enter the high-precision cosmology era, thanks also to the extraordinary and unprecedented number of galaxy clusters we will be able to identify. Clusters of galaxies are today the most massive gravitationally bound structures, with sizes of Mega-parsec (Mpc), that is, of the order of million light years. They contain up to hundreds of galaxies and can reach masses up to a quadrillion solar masses, that is, 1015 M⊙.
Following the standard model of structure formation, clusters of galaxies formed from primordial perturbations of the density field [20, 21] and are excellent laboratories to study galaxy evolution in extreme conditions as well as to perform cosmological studies [24]. Both the abundance of galaxy clusters and their spatial distribution, evaluated by means of their clustering properties, as a function of their mass and cosmic time, are strongly dependent on the dark matter and energy contents of the Universe and can be thus used as cosmological tools [25].
Based on current predictions and expectations, Euclid surveys will discover and characterize tens of thousand clusters up to early epochs of cluster formation, up to about 10 Gyr ago. The combination of cluster counts and cluster clustering will then enable us to constrain cosmological parameters in a manner that is complementary and competitive with that of the primary probes.
With the final goal to use galaxy clusters as cosmological probes, through a series of cluster finding challenges, the Galaxy Clusters Science Working Group (SWG) has selected two algorithms to detect galaxy clusters in the forthcoming Euclid surveys [2, 11, 28]. The selection of these algorithms was done upon their performance in terms of purity and completeness, and the fact that the two codes employ two complementary strategies in finding clusters.
Both codes have been validated with wide-field cosmological simulations and confirmed to yield high purity and completeness. A number of additional algorithms have been selected to assess iteratively several additional cluster properties, which are key for cosmological and galaxy evolution studies in cluster environments [1, 4]. These properties include: cluster memberships, richness, cluster profiles, refined cluster redshifts, velocity dispersions. The application of the two official cluster finders and the above-mentioned series of post-analysis algorithms will yield an official catalog of Euclid clusters as well as a number of pre-launch key project papers. These are the result of several years of work within the Galaxy Clusters SWG and constitute the benchmark of the cluster pipeline, in preparation of the upcoming work that will be performed on the real Euclid data, as soon as they will become available for scientific exploitation.
3. Galaxy evolution in clusters
As galaxy clusters are among the most massive structures in the Universe, with a large number of galaxies contained within Mpc-scale volumes, they are excellent sites to study galaxy evolution in extreme conditions. It has indeed been known that galaxy clusters can influence stellar and star formation properties of galaxies via a number of environmental driven mechanisms such as gas stripping due to the passage of the galaxies at high velocity through the hot intra-cluster medium (ICM) gas [16], galaxy-galaxy high-speed encounters with the associated tidal phenomena [5, 14], starvation due to lack of gas supply [3], as well as phenomena such as flows of cooling gas and active galactic nucleus (AGN) feedback, both ultimately associated with the brightest elliptical galaxies at the center of clusters [13]. The last ones are due to the complex interaction, known as radio-mode AGN feedback, between the ICM cooling which ultimately powers nuclear AGN activity, the accretion of super massive black holes at the center of massive ellipticals, and the launch of powerful radio jets which can reach large distances and interact with the ICM up to the cluster periphery. Euclid will be able to probe all environments in which galaxies are found, from the lowest up to the highest local densities, up to the highest redshifts. This will be possible thanks to the wide area coverage and the unprecedented survey depth of Euclid.
We will thus use Euclid data to reconstruct the full range of galaxy density field, from the lowest to the highest values, ranging from low density voids, filaments, to groups, clusters, and large-scale non-virialized proto-clusters (e.g., [6, 26]). This will be achieved by applying a number of codes and strategies that are complementary to the official cluster finder algorithms, mentioned above. In parallel, the combination of spectro-photometric Euclid data with ancillary ground and space-based ones will allow us to measure stellar and star formation properties of galaxies, including stellar masses, colors, luminosities, starformation rates, and metallicities [9]. By comparing galaxy and environment properties we will thus shed light on the co-evolution between the two up to the largest cosmic distances.
4. Summary of the conference in Bologna
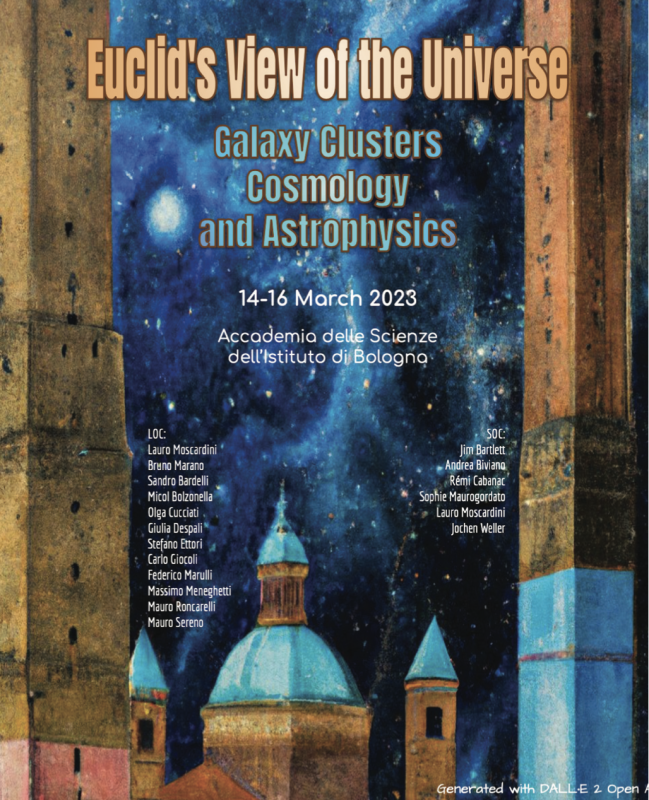
Fig. 3. Poster of the annual joint galaxy cluster SWG & OU- LE3 meeting in Bologna. Courtesy of Micol Bolzonella.
During the week of March 14-16, 2023 we had the first annual meeting3 of the galaxy cluster SWG, together with the Organization Unit Level 3 (OU-LE3) Work Package of the Euclid Science Ground Segment (Fig. 3). The latter comprises Euclid researchers which are responsible for the implementation and validation of several algorithms (i.e., processing functions) which aim at the detection and characterization of Euclid clusters and altogether represent the full Euclid cluster pipeline. This joint meeting was held in Bologna at the historical Palazzo Poggi, the main building of the Alma Mater Studiorum University of Bologna. The conference saw the active participation of 88 researchers working in Euclid on galaxy clusters, either online or in person. During the three days of the conference several talks and discussions were held, concerning the different activities of the SWG work packages. After a welcoming talk on Monday morning, over the week there were talks reporting the updates on the different WP activities concerning cluster sample selection, weak-lensing cluster mass modeling, the likelihood for cosmological studies with Euclid clusters, statistics on cluster samples, cluster mass versus observable relations, validation of Euclid cluster, the astrophysics of clusters of galaxies, cluster simulations and protoclusters. The status of the ongoing associated key project papers has also been presented by the lead authors. These papers, which are key for the success of the Euclid mission, describe the different steps, procedures, algorithms, theoretical prescriptions, and analyses that will be undertaken in the forthcoming months on real Euclid data, for their optimal exploitation concerning galaxy cluster science.
An important moment of the conference has been a joint online session with the working group on galaxy clusters of the extended ROentgen Survey with an Imaging Telescope Array (eROSITA) collaboration, which was having a parallel meeting in Germany. eROSITA is the primary instrument on-board the Russian-German Spectrum-Roentgen-Gamma space observatory [22], which was successfully launched on July 13, 2019 and is orbiting around the L2 point since then. At the end of its > 7 years mission, eROSITA will complete the first all-sky survey in the medium energy X-ray range up to 10 keV with an unprecedented spectral and angular resolution, thus allowing the detection of hot X-ray emitting plasma of ∼ 105 clusters out to redshifts z ≳ 1 [15, 18]. These eROSITA clusters, with their associated X-ray properties, are thus complementary to those the joint use of Euclid and ground-based facility will detect at optical-infrared wavelengths. During our meeting in Bologna, we discussed possible joint strategies between the eROSITA and Euclid groups working on galaxy clusters, to better exploit the complementarity of the cluster samples detected with the two missions, and thus increase the potential of galaxy clusters for cosmological studies.
References
1. Andreon, S. Richness-based masses of rich and famous galaxy clusters. A&A 2016, 587, A158. doi: 10.1051/0004-6361/201526852. arXiv: 1601.06912 [astro-ph.CO].
2. Bellagamba, F.; et al. AMICO: optimized detection of galaxy clusters in photometric surveys. MNRAS 2018, 473 (4), 5221-5236. doi: 10.1093/mnras/stx2701. arXiv: 1705.03029 [astro-ph.CO].
3. Boselli, A.; et al. Quenching of the star formation activity in cluster galaxies. A&A 2016, 596, A11. doi: 10.1051/0004-6361/201629221. arXiv: 1609.00545 [astro-ph.GA].
4. Castignani, G.; and Benoist, C. A new method to assign galaxy cluster membership using photometric redshifts. A&A 2016, 595, A111. doi: 10.1051/0004-6361/201528009. arXiv: 1606.08744 [astro-ph.CO].
5. Castignani, G.; et al. Molecular gas in two companion cluster galaxies at z = 1.2. A&A 2018, 617, A103. doi: 10.1051/0004-6361/201832887. arXiv: 1806.01826 [astro-ph.GA].
6. Contarini, S.; et al. Euclid: Cosmological forecasts from the void size function. A&A 2022, 667, A162. doi: 10.1051/0004-6361/202244095. arXiv: 2205.11525 [astro-ph.CO].
7. Cropper, M.; et al. VIS: the visible imager for Euclid. Space Telescopes and Instrumentation 2016: Optical, Infrared, and Millimeter Wave. MacEwen, H.A., et al. Eds.; Vol. 9904. Society of Photo-Optical Instrumentation Engineers (SPIE) Conference Series. July 2016, 99040Q, 99040Q. doi: 10.1117/12.2234739. arXiv: 1608.08603 [astro-ph.IM].
8. Euclid Collaboration: Lepori, F.; et al. Euclid preparation. XIX. Impact of magnification on photometric galaxy clustering. A&A 2022, 662, A93. doi: 10.1051/0004-6361/202142419. arXiv: 2110.05435 [astro-ph.CO].
9. Euclid Collaboration: Bisigello, L.; et al. Euclid preparation. XXII. Selection of quies- cent galaxies from mock photometry using machine learning. A&A 2023, 671, A99. doi: 10.1051/0004-6361/202244307. arXiv: 2209.13074 [astro-ph.IM].
10. Euclid Collaboration: Martinet N.; et al. Euclid preparation. IV. Impact of undetected galaxies on weak-lensing shear measurements. A&A 2019, 627, A59. doi: 10.1051/0004- 6361/201935187. arXiv: 1902.00044 [astro-ph.CO].
11. Euclid Collaboration: Adam, R.; et al. Euclid preparation. III. Galaxy cluster detection in the wide photometric survey, performance and algorithm selection. A&A 2019, 627, A23. doi: 10.1051/0004-6361/201935088. arXiv: 1906.04707 [astro-ph.CO].
12. Euclid Collaboration: Scaramella R.; et al. Euclid preparation. I. The Euclid Wide Survey. A&A 2022, 662, A112. doi: 10. 1051/0004-6361/202141938. arXiv: 2108.01201 [astro-ph. CO].
13. Fabian, A.C. Observational Evidence of Active Galactic Nuclei Feedback. ARAA 2012, 50, 455-489. doi: 10.1146/annurevastro-081811-125521. arXiv: 1204.4114 [astro-ph.CO].
14. Gnedin, O.Y. Tidal Effects in Clusters of Galaxies. ApJ 2003, 582.1, 141-161. doi: 10.1086/344636. arXiv: astro-ph/0302497 [astro-ph].
15. Hofmann F.; et al. eROSITA cluster cosmology forecasts: Cluster temperature substructure bias. A&A 2017, A118, 606. doi: 10.1051/0004-6361/201730742. arXiv: 1708.05205 [astro- ph.CO].
16. Jáchym P.; et al. ALMA Unveils Widespread Molecular Gas Clumps in the Ram Pressure Stripped Tail of the Norma Jellyfish Galaxy. ApJ 2019, 883 (2), 145. doi: 10.3847/1538-4357/ ab3e6c. arXiv: 1905.13249 [astro-ph.GA].
17. Laureijs R.; et al. Euclid Definition Study Report. arXiv e-prints 2011, arXiv:1110.3193. doi: 10.48550/arXiv.1110.3193 [astro-ph.CO].
18. Liu A.; et al. The eROSITA Final Equatorial-Depth Survey (eFEDS). Catalog of galaxy clus- ters and groups. A&A 2022, 661, A2. doi: 10.1051/0004-6361/202141120. arXiv: 2106.14518 [astro-ph.CO].
19. Maciaszek T.; et al. Euclid near infrared spectrometer and photometer instrument flight model presentation, performance, and ground calibration results summary. Space Telescopes and Instrumentation 2022: Optical, Infrared, and Millimeter Wave; Coyle, L.E.; Matsuu- ra, S.; and Perrin, M.D. Eds.; Vol. 12180. Society of Photo-Optical Instrumentation Engi- neers (SPIE) Conference Series. Aug. 2022, 121801K. doi: 10.1117/12.2630338. arXiv: 2210.10112 [astro-ph.IM].
20. Peacock, J.A. Cosmological Physics. Cambridge University Press: Cambridge, 1999.
21. Peebles, P.J.E. Principles of Physical Cosmology. Princeton University Press: Princeton, 1993. doi: 10.1515/9780691206721.
22. Predehl, P.; et al. The eROSITA X-ray telescope on SRG. A&A 2021, 647, A1. doi: 10.1051/0004-6361/202039313. arXiv: 2010.03477 [astro-ph.HE].
23. Racca, G.D.; et al. The Euclid mission design. Space Telescopes and Instrumentation 2016: Optical, Infrared, and Millimeter Wave; MacEwen, H.A.; et al. Eds.; Vol. 9904. Society of Photo-Optical Instrumentation Engineers (SPIE) Conference Series. July 2016, 99040O. doi: 10.1117/12.2230762. arXiv: 1610.05508 [astro-ph.IM].
24. Rhodes, J.; et al. Scientific Synergy between LSST and Euclid. ApJS 2017, 233 (2), 21. doi: 10.3847/1538-4365/aa96b0. arXiv: 1710.08489 [astro-ph.IM].
25. Sartoris, B.; et al. Next generation cosmology: constraints from the Euclid galaxy cluster survey. MNRAS 2016, 459 (2), 1764-1780. doi: 10.1093/mnras/stw630. arXiv: 1505.02165 [astro-ph.CO].
26. Sousbie. T. The persistent cosmic web and its filamentary structure – I. Theory and imple- mentation. MNRAS 2011, 414 (1), 350-383. doi: 10.1111/j.1365-2966.2011.18394.x. arXiv: 1009.4015 [astro-ph.CO].
27. Tutusaus, I.; et al. Euclid: The importance of galaxy clustering and weak lensing cross-cor- relations within the photometric Euclid survey. A&A 2020, 643, A70. doi: 10.1051/0004- 6361/202038313. arXiv: 2005.00055 [astro-ph.CO].
28. Werner, S.V.; et al. S-PLUS DR1 galaxy clusters and groups catalogue using PzWav. MNRAS 2023, 519 (2), 2630-2645. doi: 10.1093/mnras/stac3273. arXiv: 2211.04243 [astro-ph. GA].
Footnotes
1 https://www.spacex.com/launches/mission/?missionId=euclid.
2 All images are in full-color in the online edition of the Annales
3 https://sites.google.com/inaf.it/galaxyclusterseuclidbo2023.